Study Notes for Membrane Transport (For making your own liposomal sprays)
By Dennis Klocek 9 min read
Liposomes can trap both hydrophobic and hydrophilic substances and unstable compounds (for example, antimicrobials, antioxidants, flavors and bioactive elements).
Proteins creating transport channels across phospholipid membranes, mitochondrial and chloroplast inner membranes require more proteins for transport. (Egg yolks have proteins as well as lipids. Egg lecithin is useful for production of liposomes it produces more permeable liposomal bilayer having both protein and lipids and lecithin).
Terpenes (especially sesquiterpenes) in the mix aid in membrane transport penetration.
Fatty acids / salts (soaps) form micelles, not liposomes. Avoid ionic and anionic surfactants (detergents) in sonication – however yucca extract is a non-ionic surfactant, low concentrations in a mix aids in membrane transport penetration.
Rate of surfactant in most effective antibacterial liposome formation is 50 micrograms per milliliter of water
- 50 micrograms in one drop
- 20 drops in one milliliter
- rate of surfactant is one drop per twenty drops
- one teaspoon is ~ 100 drops
- rate is then 5 drops per teaspoon
- one quart is ~ 200 teaspoons
- 10 teaspoons per quart
- 3 teaspoons in a tablespoon
About three tablespoons / quart of stock solution (ferment) was surfactant dilution rate for non ionic surfactants for penetration of bacterial membranes.
For plants this might be dialed back to two tablespoons per quart of stock ferment solution for penetrating plant cell membranes. For spray the stock solution would then be sonicated in small amounts and refrigerated to be added to a quart of water at the rate of two tablespoons per quart.
Tutorial: Making your own liposomal EOs
Liposomal encapsulation technology (LET) is the newest delivery technique used by medical investigators to transmit drugs that act as curative promoters to the assured body organs. This form of delivery system proposal targeted the delivery of vital combinations to the body. LET is a method of generating sub-microscopic foams called liposomes, which encapsulate numerous materials. These ‘liposomes’ form a barrier around their contents, which is resistant to enzymes in the mouth and stomach, alkaline solutions, digestive juices, bile salts, and intestinal flora that are generated in the human body, as well as free radicals. The contents of the liposomes are, therefore, protected from oxidation and degradation. This protective phospholipid shield or barrier remains undamaged until the contents of the liposome are delivered to the exact target gland, organ, or system where the contents will be utilized. Colloidal particulates result from the physical incorporation of a drug into a particulate colloidal system, among these colloidal properties liposomes have been most studied. Their attractiveness lies in their composition, which makes them biodegradable and biocompatible. Liposome involves an aqueous core entrapped by one or more bilayers composed of natural or synthetic lipids. They are composed of natural phospholipids that are biologically inert and feebly immunogenic, and they have low inherent toxicity.
Although liposomes are like biomembranes, they are still foreign objects of the body. Therefore, liposomes are known by the mononuclear phagocytic system (MPS) after contact with plasma proteins. Accordingly, liposomes are cleared from the blood stream. These stability difficulties are solved through the use of synthetic phospholipids, particle coated with amphipathic polyethylene glycol, coating liposomes with chitin derivatives, freeze drying, polymerization, microencapsulation of gangliosides [17].
This study evaluates the ability of the terpenes incorporated in liposomes on the in vitro skin delivery of hydrophobic model drug, nimesulide (NE). To this purpose, so-called terpene liposomes (TPs), which are composed of phospholipid and three types of terpene, citral, limonene and cineole. The obtained formulations were characterized in terms of size distribution, zeta potential and morphology. The efficiency of TPs on skin delivery of NE was studied using in vitro Franz diffusion cells and abdominal rat skin in comparison with conventional liposomes and ethanolic solutions of NE. Results showed that all the used TPs had spherical structures with negative zeta potential, low polydispersity (PDI < 0.2), nanometric size range (z-average no more than 150 nm). TPs improved the entrapment efficiency (EE%) and gave good physical stability. In vitro skin permeation data showed that TPs were able to give a significant improvement of NE permeation through the rat skin in comparison with conventional liposomes and drug solution. Moreover, the TPs prepared with limonene were also able to deliver a higher amount of NE than the other formulation, thus suggesting that NE delivery to the skin was strictly correlated to type of terpenes incorporated liposomes.
Study: TRANSFEROSOMES: A CARRIER FOR DRUG DELIVERY – World Journal of Pharmaceutical and Life Sciences (PDF)
The term Transfersome and the underlying concept were introduced in 1991 by Gregor Cevc. Numerous groups have since been working with similar carriers, frequently using different names (e.g., elastic vesicle, flexible vesicle, etc.) to describe them.
In a broader sense, a Transfersome is a highly adaptable, stress-responsive complex aggregate. The form preferred by researchers and pharmacologists is an ultradeformable vesicle possessing an aqueous core surrounded by the complex lipid bilayer.
Interdependencies inherent in the local composition and shape of the bilayer makes the vesicle both self-regulating and self-optimizing. This enables the Transfersome to cross various transport barriers efficiently, and then act as a Drug carrier for non-invasive targeted drug delivery and sustained release of therapeutic agents.
Composition and mechanism of action
The carrier aggregate is composed of at least one amphipathic (such as phosphatidylcholine), which in aqueous solvents self-assembles into a lipid bilayer that closes into a simple lipid vesicle. By addition of at least one bilayer softening component (such as a biocompatible surfactant or an amphiphile drug) lipid bilayer flexibility and permeability are greatly increased. The resulting Transfersome is optimized for flexibility and permeability, and can therefore adapt its shape to ambient conditions easily and rapidly by adjusting local concentration of each bilayer component to the local stress experienced at the bilayer. Since its basic organization is broadly similar to a liposome, a Transfersome differs from more conventional vesicles primarily by its “softer”, more deformable, and better adjustable artificial membrane.
Another beneficial consequence of strong bilayer deformability is the increased affinity of a Transfersome to bind and retain water. An ultradeformable and highly hydrophilic vesicle always tends to avoid dehydration, which may involve a transport process related to, but not identical with forward osmosis. For example, a Transfersome vesicle applied on an open biological surface, such as non-occluded skin, tends to penetrate its barrier and migrate into the water-rich deeper strata to secure adequate hydration. Barrier penetration involves reversible bilayer deformation, but must not compromise either vesicle integrity or barrier properties for the underlying hydration affinity and gradient to remain unimpaired.
Since it is too large to diffuse through the skin, the Transfersome must find and exploit a suitable route through the organ. Use of Transfersome vesicles for drug delivery therefore relies on the carrier’s ability to widen and overcome the hydrophilic pores in the skin or some other (e.g. plant cuticle) opening. The subsequent gradual release of the active agent from the drug carrier allows the drug molecules to diffuse and finally bind to their targets. Drug transport to an intra-cellular action site may also involve fusion of the carrier’s lipid bilayer with the cell membrane, unless the vesicle is taken up actively by the cell in the process called endocytosis.
Gases, such as O2 and CO2, and small, uncharged polar molecules, such as urea and ethanol, can readily move by passive (simple) diffusion across an artificial membrane composed of pure phospholipid or of phospholipid and cholesterol.
The hydrophobicity of a substance is measured by its partition coefficient K, the equilibrium constant for its partition between oil and water. The higher a substance’s partition coefficient, the more lipid-soluble it is. The first and rate-limiting step in transport by passive diffusion is movement of a molecule from the aqueous solution into the hydrophobic interior of the phospholipid bilayer, which resembles oil in its chemical properties. This is the reason that the more hydrophobic a molecule is, the faster it diffuses across a pure phospholipid bilayer. If a transported substance carries a net charge, its movement is influenced by both its concentration gradient and the membrane potential, the electric potential (voltage) across the membrane. The combination of these two forces, called the electrochemical gradient, determines the energetically favorable direction of transport of a charged molecule across a membrane. The electric potential that exists across most cellular membranes results from a small imbalance in the concentration of positively and negatively charged ions on the two sides of the membrane. Very few molecules and no ions can cross a pure phospholipid bilayer at appreciable rates by passive diffusion. Thus transport of most molecules into and out of cells requires the assistance of specialized membrane proteins. By forming a protein-lined pathway across the membrane, transport proteins are thought to allow movement of hydrophilic substances without their coming into contact with the hydrophobic interior of the membrane.
ATP-powered pumps (or simply pumps) are ATPases that use the energy of ATP hydrolysis to move ions or small molecules across a membrane against a chemical concentration gradient or electric potential or both. This process, referred to as active transport, is an example of a coupled chemical reaction. In this case, transport of ions or small molecules “uphill” against an electrochemical gradient, which requires energy, is coupled to the hydrolysis of ATP, which releases energy. The overall reaction—ATP hydrolysis and the “uphill” movement of ions or small molecules—is energetically favorable. Channel proteins transport water or specific types of ions and hydrophilic small molecules down their concentration or electric potential gradients. Such protein-assisted transport sometimes is referred to as facilitated diffusion. Channel proteins form a hydrophilic passageway across the membrane through which multiple water molecules or ions move simultaneously, single file at a very rapid rate.
CO2 and steroids are active in passive diffusion with little energy loss.
Glucose and amino acids facilitate transport through diffusion.
Lipids and small hydrophilic molecules (glucose sucrose) interact with the lipid elements in the membrane with much more transport when they are protein mediated.
The lumen of plant vacuoles is much more acidic (pH 3 to 6) than is the cytosol (pH 7.5). The acidity of vacuoles is maintained by a V-class ATP powered proton pump (see Figure 7-6) and by a PPi-powered pump that is unique to plants. Both of these pumps, located in the vacuolar membrane, import H ions into the vacuolar lumen against a concentration gradient. The vacuolar membrane also contains Cl and NO3 channels that transport these anions from the cytosol into the vacuole. Entry of these anions against their concentration gradients is driven by the inside-positive potential generated by the H pumps. The combined operation of these proton pumps and anion channels produces an inside-positive electric potential of about 20 mV across the vacuolar membrane and also a substantial pH gradient.
The proton electrochemical gradient across the plant vacuole membrane is used in much the same way as the Na electrochemical gradient across the animal-cell plasma membrane: to power the selective uptake or extrusion of ions and small molecules by various antiporters.
In the leaf, for example, excess sucrose generated during photosynthesis in the day is stored in the vacuole; during the night the stored sucrose moves into the cytoplasm and is metabolized to CO2 and H2O with concomitant generation of ATP from ADP and Pi. A proton/sucrose antiporter in the vacuolar membrane operates to accumulate sucrose in plant vacuoles. The inward movement of sucrose is powered by the outward movement of H, which is favored by its concentration gradient (lumen cytosol) and by the cytosolic-negative potential across the vacuolar membrane (see Figure 7-23). Uptake of Ca2 and Na into the vacuole from the cytosol against their concentration gradients is similarly mediated by proton antiporters.
Unlike animal cells, plant, algal, fungal, and bacterial cells are surrounded by a rigid cell wall. Because of the cell wall, the osmotic influx of water that occurs when such cells are placed in a hypotonic solution (even pure water) leads to an increase in intracellular pressure but not in cell volume. In plant cells, the concentration of solutes (e.g., sugars and salts) usually is higher in the vacuole than in the cytosol, which in turn has a higher solute concentration than the extracellular space. The osmotic pressure, called turgor pressure, generated from the entry of water into the cytosol and then into the vacuole pushes the cytosol and the plasma membrane against the resistant cell wall. Cell elongation during growth occurs by a hormone-induced localized loosening of a region of the cell wall, followed by influx of water into the vacuole, increasing its size.
Resources
- Transferosomes – A vesicular transdermal delivery system for enhanced drug permeation
- TRANSFEROSOMES: A CARRIER FOR DRUG DELIVERY – World Journal of Pharmaceutical and Life Sciences (PDF)
- Effect of terpene liposomes on the transdermal delivery of hydrophobic model drug, nimesulide: Characterization, stability and in vitro skin permeation
- Membrane Transport, 2
- Molecular Cell Biology
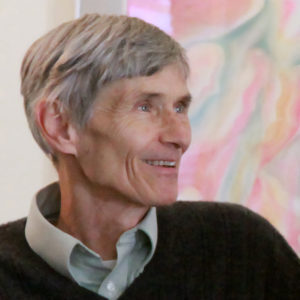
Dennis Klocek
Dennis Klocek, MFA, is co-founder of the Coros Institute, an internationally renowned lecturer, and teacher. He is the author of nine books, including the newly released Colors of the Soul; Esoteric Physiology and also Sacred Agriculture: The Alchemy of Biodynamics. He regularly shares his alchemical, spiritual, and scientific insights at soilsoulandspirit.com.
Similar Writings
Tuesday of Holy Week
The theme is Tuesday of Holy Week. Monday is the cursing of the fig tree. Tuesday is when there is a kind of turning. Tuesday is Mars day. Mars is aggression. There is a war of words that Christ was having with the Pharisees because he was out in the countryside preaching. He was cutting…
Working with Symbolic Images
ALCHEMICAL EARTH CONSCIOUSNESS Alchemically, learning requires that there be a process of soul breathing , that is the capacity to form accurate inner pictures that we can follow as they change inside of us. The process of finding and then refining inner pictures is a form of breathing that can be cultivated into imagination. Exercise:…